In my second to last article I explained that, deep down, light consists of energy packets – quanta. These are created, for example, when light interacts with atoms, the building blocks of our world. That sounds very daring and raises many questions, some of them deeply philosophical. And while you are racking your brains about it, one question arises: who actually thought up this up?
My last article was dedicated to Max Planck and the black body. He was able to explain the colour distribution of thermal radiation with the help of the assumption that the body can only emit energy in packets. But Planck dismissed this as a mathematical trick and did not think about its consequences – that he had contradicted classical physics – let alone recognise its relevance.
So the question is: who else can be considered as the father of quantum mechanics?
None other than Albert Einstein. Although most people probably associate him more with the theory of relativity, Einstein made a significant contribution to the development of quantum physics. In fact, he received his Nobel Prize in 1921 not for the theory of relativity, but for his explanation of the photoelectric effect – one of the key experiments in quantum physics. And like many discoveries in physics, this one was pure chance.
Today we know that light is electromagnetic radiation, i.e. temporally and spatially oscillating electric and magnetic fields. In the 19th century, however, this was not yet known. In 1864, James Clerk Maxwell predicted the existence of such waves and also determined on the spot the speed at which they propagate: the speed of light. Incidentally, this is exactly 299,792,458 m/s – no decimal point, no measurement inaccuracy. This is because the unit of length, the metre, is defined by the speed of light:
A metre is the distance that light travels in vacuum in 1/299,762,458 seconds.
So how long a metre actually is depends on the speed of light. This is because the speed of light is a fundamental physical constant – it is therefore exactly the same everywhere in the universe and forever. It is the highest speed that anything can have. And 299,792,458 m/s is pretty fast. I’ll try to illustrate this with a little anecdote.
Roadtrip to Andromeda
I once watched a documentary about astrophysics with someone in the evening when there was nothing better on TV. They said our neighbouring galaxy Andromeda is just 2.5 million light years away. You could almost wave to each other! The other person couldn’t imagine much about that and asked me, “How long would it take to get there by car?”
Good question, no idea. Let’s do the math!
So, a light year is a unit of measurement for a distance – not time, as you might mistakenly think. Since distances in astrophysics are very large (it’s no coincidence that we say something is astronomically large), they are often expressed in terms of the speed of light. A light year is the distance that light travels in one year. The definition is very similar to that of the metre – in the same spirit, you could also call the metre a 1/299,792,458th light second. But that’s a bit unwieldy, so we’ll say metre.
For astronomical distances, however, the light year is quite handy because it makes the numbers smaller. The sun, for example, is 8 light minutes away. That means it takes light 8 minutes to get from the sun to us. So if the sun were to disappear right now, we wouldn’t notice it for 8 minutes. That might sound like a long time, but considering how far away the sun is, that’s super fast!
Using the speed of light, we can work out that 8 light minutes is about 150 million kilometres. If we now travel very fast, say 200 km/h, it will take us a good 85 years. So, with a healthy lifestyle, that can actually be done in a lifetime! Leaving aside such practical details as the lack of roads, petrol stations, air and the temperatures on the way (I’m a theoretical physicist, so neglecting such things is more or less my job).
Andromeda, however, is now a little further. Namely 2.5 million light years, that is km (a 2 with 19 zeros). So you’re a whopping 13,500 billion years on the road.
“That’s supposed to be close?” I was asked in shock. “These astrophysicists are crazy!”
In their defence, on astronomical scales that is indeed small. The largest dimension we can imagine is the size of the universe. You can actually estimate that: We know with astonishing accuracy how old the universe is, namely 14 billion years (if you’ve been paying attention, you know that from the Big Bang Theory intro music). In the beginning, the baby universe was compressed into a single point. During the Big Bang, a lot of stuff flew out of this point, such as radiation, which, as we now know, spreads at the speed of light. Since nothing can be faster than light, the diameter of the universe can therefore be a maximum of billion light years.
Let’s say one end of the universe is in Constance and the other in Flensburg, i.e. once across Germany. That’s about 1000 km. Then Andromeda is just 180 m away from me, the Milky Way – you can comfortably walk across in 2 minutes and actually wave to each other.
Jumping sparks
Enough of our excursion into other galaxies and let’s get back to the electromagnetic radiation that Maxwell predicted. In 1864, this was purely Maxwell’s conjecture and had not been proven. It was Heinrich Hertz who took it upon himself to back up this hypothesis with experiments.

The experiment he thought up involved a sparkover between two electrodes. Some of you might have seen this in physics class: Two electrodes, usually two metal balls, are placed in close proximity – but they do not touch. A high voltage is applied between the two and when the voltage is high enough, a spark jumps over. This is because the insulating material between the electrodes (i.e. the air) briefly becomes conductive due to the high voltage, allowing the electrons to jump from one electrode to the other.
Hertz was interested in precisely this spark, but had problems seeing it properly in daylight. So he packed the experiment in a box and looked at the spark through a glass window. At least that was the idea, because he noticed that the spark in the box had suddenly become much smaller. Since the box was the only difference, it had to be because of that – the light obviously had an effect on the spark!
Apparently this was not exciting enough for Hertz because he returned to his original experiment with which he wanted to prove the existence of electromagnetic radiation – which he actually succeeded in doing in 1886. This discovery is considered a great triumph of the 19th century. For which, by the way, there was no Nobel Prize, in case anyone was wondering, because this was only founded in 1901.
But we are more interested in the triumphs of the 20th century anyway. Fortunately, Hertz’s assistant at the time, Wilhelm Hallwachs, took up the project and pursued it further. He discovered that the UV radiation in sunlight caused the spark to jump over more easily. The type of light seemed to be important, because it didn’t work with a torch that had no UV component. It also depended on the metal itself – it worked better with some materials than others. Moreover, this effect weakened over time – the metal seemed to wear out. Even though the observations were not yet understood, the effect was named the Hallwachs effect in Hallwachs’ honour.
The discovery of photons
In 1905, 5 years after Planck’s solution of the black body problem, Einstein took up the Hallwachs effect and tried to understand it. In this respect, he was very much like Planck: he did not carry out any experiments himself, but tried to explain the experiments of other physicists.
You have to remember that at Einstein’s time, neither photons nor electrons were known. All that was known was that a metal loses its negative charge when it is irradiated with light. But why? A tough nut to crack!
Einstein was obviously inspired by Planck in his attempt to solve the problem. Planck had discovered that the black body can only emit energy in certain packets. The frequency f of the emitted light is proportional to the released energy E, or E = h f for short, with Planck’s quantum of action h named after Planck.
Einstein then thought: Why shouldn’t this apply to light in the same way? With this simple conclusion, the light quantum hypothesis was born: light consists of packets – later named photons – whose energy is proportional to their frequency. That was all it took to explain the Hallwachs effect, now called the photoelectric effect:
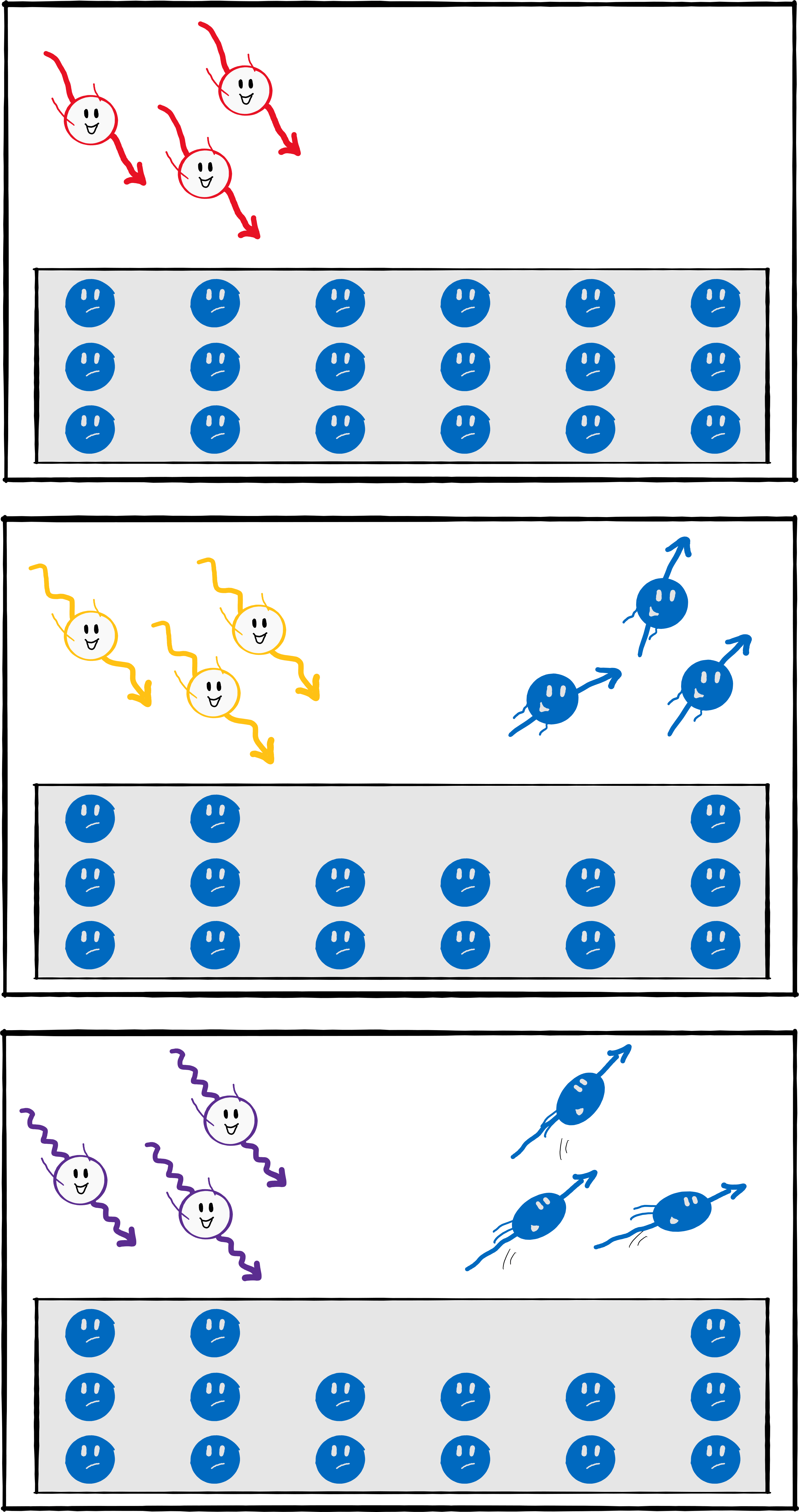
There are electrons in the metal, i.e. small particles that carry a small amount of negative charge. In fact, the smallest possible amount of electrical charge – it is also called the elementary charge. To release an electron from the metal, you need a certain minimum amount of energy which depends on the material. If you shine light at a frequency that is too low (for example, red light instead of UV), the energy of the light packets is not sufficient to release an electron. But if you increase the frequency/energy above a certain value, the energy is sufficient to release electrons. If you increase the frequency even more, there is even some energy left over that the electron can use to speed up. The higher the frequency/energy of the light, the faster the electron. The relationship is linear: if you double the frequency, you also double the speed.
The intensity of the light has no influence on the speed of the electrons but it does on the amount of electrons released. The stronger the light the more charge is released.
Quantum mechanics – the unwanted child
Even when Einstein spoke of quantisation alongside Planck, this idea was far from being accepted. In contrast to Planck, Einstein no longer even presented it as a mathematical trick but actually claimed that light was a stream of particles.
That was difficult to accept. There were so many experiments and also one’s own everyday experience that showed that light behaved like a wave. Was that suddenly all wrong? Yes and no, because light does indeed behave like both, which is called wave-particle duality. But that is a topic for another time.
Some physicists, such as Robert Milikan, were particularly disturbed by the particle picture of light. He found this idea so absurd that he spent all his energy trying to disprove Einstein. We remember Boltzmann and Planck – rivalry is fertiliser for scientific progress! In fact, his experiments did not refute Einstein’s hypothesis but supported it – at least mathematically. He still did not believe in the actual existence of photons.
Einstein himself also struggled with his discovery. But he did not go so far as to call it a mathematical trick. Although he contributed a lot to the discovery of quantum physics, he resisted and doubted it for a long time. In fact, until the end of his life, he was convinced that quantum physics was incomplete.
Quantum physics was officially recognised in 1911, six years after Einstein’s discovery, with the Solvay Conference on “Radiation Theory and Quanta”. The fact that quantum physics was openly mentioned in the title of a conference and thus given a place was groundbreaking. Everybody saw that quantum theory would change the course of physics.
And they were right. The discovery of quantum mechanics was the great breakthrough of the 20th century. And today it is as present as ever. News of new discoveries and advances in quantum technology are regularly in the news, quantum mechanics has entered the consciousness of the broad population, we are on the threshold of a new age. Let me take you into a world where everything is different from what you are used to; that is more reminiscent of Alice’s Wonderland than of dry physics lessons at school; and that has pushed even the greatest geniuses of the 20th century to the limits of their imagination.
Welcome to the quantum world!
Do you like what you read? If you don’t want to miss any new posts, don’t forget to subscribe to my blog.
Sources:
https://universaldenker.de/physik/photoeffekt/geschichte [Not available anymore]