In my last article, I explained that, deep down, light consists of energy packets – quanta. These are created, for example, when light interacts with atoms, the building blocks of our world. That sounds very daring and raises many questions, some of them deeply philosophical. And while you are racking your brains about it, one question arises: Who actually thought this up?
A very good question that has no clear answer. However, there are two strong positions that I would like to present here. Let me elaborate:
“Our story begins in ancient Greece. It is a warm summer evening around 600 before Christ, you are shopping at the market, or the Agora.”
Just kidding, not quite that far. Let’s fast-forward to the end of the 19th century: Alexander Graham Bell invented the predecessor of the smartphone, the French built and railed against the Eiffel Tower and Tolstoy mused about war and peace. In these exciting times, things also got turbulent in physics. The world split into two camps and at the centre of their excitement: entropy.
Chaos, cards, and cold coffee
If the word entropy means nothing to you, you are probably not alone. You might conclude that it has little influence on our everyday lives. But far from it: entropy is everywhere! It belongs to the realm of thermodynamics and is, in short, a measure of chaos. Now it sounds much more exciting, doesn’t it?
The meaning of entropy is best explained with an example: Imagine you have a pack of cards, brand new and still packaged. Maybe it has pictures of your favourite atoms on the back. You open it and look at the cards: they are in perfect order. That means the chaos – the entropy – is minimal. If you now shuffle the cards, the chaos increases and so does the entropy. As soon as the cards are completely and perfectly shuffled, the entropy has reached its maximum value.
A principle of thermodynamics is that chaos never decreases on its own. When you were a child, did you ever “accidentally” tidy your room? Did you ever “accidentally” shuffle a pack of cards back into order? I dare say that has never happened! (If it has, please tell me your trick.) But: Why not? From a purely technical or physical point of view, it is not impossible. There is no “dark force” that brings the world into chaos.
The reason for this is not as complicated as you might think: there are many ways to mix up a pack of cards, but there is only one way to put them in order.
How many possibilities are “many”? These are the questions physicists (or mathematicians) ask themselves when they are bored. Let’s do a little exercise and remember the probability calculus (or stochastics) from our school days.
Let’s rephrase the question a little: How likely is it to randomly put a pack of cards in the right order? Let’s say you have a well-shuffled short deck with 32 cards. Let’s say the correct order we are looking for starts with the 7 of hearts. The probability that exactly this card is the first is 1/32, since we are looking for one of 32 cards. This is followed by the 8 of hearts with a probability of 1/31, since the 7 of hearts is already out and there are only 31 cards left. The next card then has a probability of 1/30 and so on and so forth.
If we multiply all these probabilities , the probability that the pack of cards happens to be correctly ordered is equal to 1/263130836933693530167218012160000000. The large number after the fraction bar indicates the number of possible combinations to arrange a pack of cards. And since we are looking for a specific one, there is a 1 before the bar. In case you have a problem naming this number (like me): There are 35 zeros after the decimal point and then there is a 4. So while the probability that the deck happens to be correctly ordered is almost zero, the probability that the deck is shuffled (which is basically all other constellations) is practically equal to one.
Very large or small numbers are difficult to imagine. Here is some help: Let’s imagine that your way of shuffling is very simple and you always put exactly one card in any other place of the deck. You repeat this once every second. With this method, you would need an average of 1026 years (that’s a one with 26 zeros behind it) to randomly shuffle the cards into the right order. That’s 10 quadrillion times as long as the age of the universe. So I’m not taking too much of a risk when I say that no one has ever shuffled a pack of cards into the right order by pure chance.
In physics, the whole thing is wrapped up in the second law of thermodynamics: the entropy, i.e. the chaos, of a system never decreases on its own. It grows, however, very easily: rooms become messy, card games get mixed up, milk and coffee get mixed. Another example: the coffee in the cup gets cold over time, as the temperature of the coffee and its surroundings equalise. But coffee will never take energy from the surroundings and suddenly heat up. Theoretically, the energy could flow either way, but practically, the heating of the coffee contradicts the second law of thermodynamics.
Atomists vs. Energeticists
I have used two very different images of entropy: One based on statistics (playing cards) and the other on the flow of energy (cold coffee). And these are exactly the two camps that were established at the end of the 19th century.
On the one hand, there is Ludwig Boltzmann, a fervent advocate of atomism. Although the idea that the world consists of tiny, indivisible building blocks has existed since the ancient world, it was not yet considered a proven fact among physicists in the 19th century. Boltzmann, however, was firmly convinced of the existence of atoms. It now follows from this assumption that the natural mixing of systems is a statistical effect: just like a pack of cards, there are simply many more ways to throw atoms around chaotically instead of lining them up neatly.
In the other corner, we find Max Planck, who belongs to the camp of the “energeticism“. He did not believe in atoms, but in the continuity of the world. Just like the energy in the coffee cup, he believed that matter could be divided into arbitrary pieces. He was convinced that the second law of thermodynamics always applies – not only in the statistical mean, as Boltzmann claimed.
What is black?
At that time, the focus of attention in thermodynamics was the black body. Physicists find many things exciting – springs, little cars on ramps, Star Wars – but why a black box? To explain this, we have to ask what black actually means in physical terms.

Light can have different colours. We see this most impressively in a rainbow. From a physical point of view, light is an electromagnetic wave, i.e. electric and magnetic fields that decrease and increase over time. We call the number of oscillations per second the frequency of light. Now colour is nothing but the frequency of light: if light has a very high frequency, it oscillates very fast and our eye perceives this as blue. If the frequency is low, we perceive it as red.
There are, roughly speaking, two different ways to see light. The more direct one is to look directly into a lamp, at a screen, or into the sun (not recommended!). The light emitted by the light source hits our eyes directly and without detours. Often, the light from direct light sources has many different frequencies that mix together to form some shade of white. Sunlight, for example. In a rainbow, these different parts are split up and we see the different frequencies neatly lined up. Therefore, white light is, when you get right down to it, “very colourful light”.
But we also see many things around us that do not glow. Like the coffee cup or the pack of cards. Have you ever wondered why we see these things at all?
Look at your coffee cup again (if you still don’t feel like having coffee, you are probably less addicted than I am). When the cup is on the table, sunlight hits it, part of the light is reflected by the ceramic, it hits our eye and due to the chemical and physical properties of the material, the cup appears (for example) red to us.
Now imagine you put your coffee cup in a cupboard and close the door. What colour is the cup now that it is in the cupboard? Is it still red?
No. The impression of colour comes from the frequency of light and there is no light in the cupboard. You might be thinking, “Why is she making such a fuss about that it’s dark in the cupboard? Does she think I’m stupid?” Absolutely not! When I first thought about it I was completely fascinated and I still am. There is no light in the cupboard, which means the cup has no colour at all.
And that is precisely the definition of “black”. It means: no ray of light comes from this object into our eye, no colour, nothing. It is the opposite of white, the opposite of “all colours”.
Objects can appear black when there is no light source anywhere, such as in the cupboard. However, if you drink your coffee without milk, it will also be black outside the cupboard – even if the sun is shining. The reason for this is that black objects, like the coffee, swallow up most of the light that hits them. Therefore, nothing remains that could be reflected and hit our eye.
A black body is therefore an object that absorbs (i.e. swallows) all the light that hits it. Unlike coffee, in which we can still see a hint of brown because a little light is still reflected, an ideal black body (i.e. the one that physicists are interested in) swallows absolutely every ray of light that hits it. And that doesn’t just mean visible light, but all radiation, such as microwaves, UV radiation or radio waves.
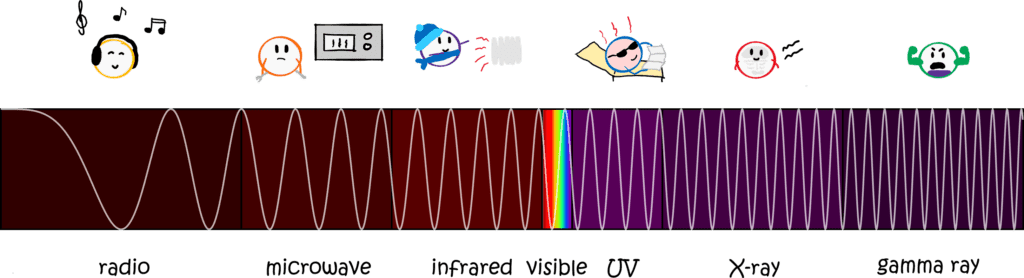
But s/he who takes it in must also be able to dish it out (that was the saying, wasn’t it?). Light is nothing but energy and, as everywhere in physics, conservation of energy also applies to black boxes. This means that the black body must radiate as much energy per second as it has swallowed. But what does the light that the black body emits look like? What colour does a black body have?
If you are thinking: black, then I am sorry to disappoint you. Black is the absence of light, which means that nothing can radiate black. At most, it can radiate nothing. But I can reassure you: the question is anything but easy to answer and has captivated physicists for a long time!
What hotplates, black boxes and blue stars have in common
In Boltzmann’s and Planck’s time, it had already been discovered that the colour of a black body depends only on its temperature. This is why this radiation is also called thermal radiation. The hotter the body, the more energy it radiates and the “bluer” the light. In fact, this is something that all of us have observed in everyday life. Think of a piece of metal (or a cooker pipe or a hot plate) that you heat up. At first it is black or silver, but eventually it starts to glow red. That is heat radiation! But even before the metal glows red, something happens. It emits light at a frequency lower than red: infrared (literally meaning below-red, very creative). People are (usually) less hot than a hotplate and therefore emit heat radiation in the infrared range. Infrared cameras can therefore be used to detect people and other warm objects.
So the metal first radiates infrared and then red. But if we heat it up even more, it will eventually glow yellow, white or even blue. Can you think of anything big that glows yellow? The sun! But it’s a little more complicated than simply saying that the sun’s heat radiation is yellow. Heat radiation does not consist of just one colour, but of many. We can calculate the average colour of sunlight from the temperature of the sun and the result is… green. I can reassure you, you do not have a yellow-green weakness. The maximum of the heat radiation is green, but there are still strong parts of red and blue in it (and also infrared and UV, so always put sunscreen!). In the end, everything mixes to a yellowish-white, as we know it.
If we increase the temperature even more, the maximum slides to blue. You can see an example of this in the sky. I don’t mean the blue of the sky, that has another reason, but stars! If you look very closely at night, you will notice that stars twinkle red, yellow or blue. From the colour of the stars, you can determine their temperature, from the temperature you can determine their age and also their life expectancy.
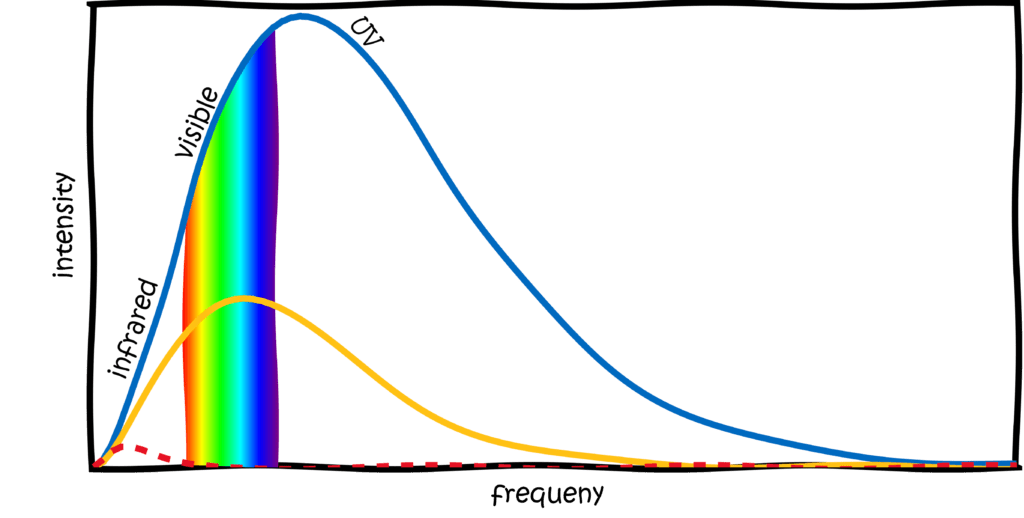
In short, thermal radiation is omnipresent, very powerful and definitely worth the attention physicists paid to it. They wanted to know exactly what colour mixture a black body emits at what temperature. It was hoped that this would provide a fundamentally better understanding of the interaction between light and matter. Because one principle of physics is: as long as you can’t describe an observation with a formula, you obviously haven’t understood it well enough.
Planck’s ordeal
It is 1896. Where are we? The physicist Wilhelm Wien has just established his empirical law of radiation, which correctly describes thermal radiation in qualitative terms. A lot of clever words to describe a lot of wishy-washy.
“Empirical” means: he made a clever guess and introduced some numbers so that his law agrees with observations. If you look at the sky and measure that 24 hours pass between two sunrises, you have empirically determined the length of a day. To do that, you don’t have to know anything about the Earth’s orbit around the Sun and the Earth’s rotation around itself. But if you did, you could determine the length of a day without having to look out of the window. “Qualitative” means that his law “approximately” agrees with observations. If I know that a day has 24 hours, I can qualitatively say that the sun must set once sometime within 24 hours – presumably sometime in the middle. But if I know the Earth’s rotation and orbit, I can quantitatively predict the exact time when the Sun will set.
Planck was not a fan of empiricism and qualitative statements. He wanted a proper derivation including a physical explanation. So he sat down and did just that, because he is Max Planck. It was in 1900 that he wrote down a better formula, which is now also quantitatively correct. But at one point in the derivation, he only made a lucky guess, and he knew it. Moreover, it was a point that concerned his beloved entropy. Although the new curve could describe the observations perfectly, Planck was still unhappy because he did not understand his own formula.
Salvation came very soon, but unfortunately not as Planck had hoped. For in order to solve the problem, he had to enter enemy territory: the field of statistics, the home of atomistics. He noticed a similarity between his problem and one of Boltzmann’s equations with which he could solve his problem. He tried to save himself from the misery by calling this circumstance a “mathematical trick”.
In this act of desperation, he had to assume that energy in the black body occurs in certain, discrete packets. The amount of energy (E) per packet depends only on the frequency (f) of the radiation:
E = h f
We have already talked about light, frequencies and energy, and here we find the crux of the matter. What is for particles with mass,
is for light. h is an auxiliary constant (the letter stems from the German translation “Hilfskonstante”) that Planck introduced and was later named Planck’s constant in his honour. This is not an empirical constant, as with Wien, but a fundamental physical constant. A constant, like the speed of light, which – you could say – is given by God. It cannot be changed by anything and is absolutely the same everywhere in the universe. If you divide it by 2π, which makes many formulas look prettier, it is represented with the symbol ℏ (pronounced: h-bar). It is contained in every formula of quantum physics and is thus the heraldic animal of quantum physics. If you ever meet someone with a ℏ tattoo (and believe me, that’s a thing), now you know what to talk to him or her about.
Planck, however, had no idea of the stir he would create when he wrote this innocent h into his formula. For him, it was a mathematical trick, a formal assumption, a necessary evil, and it was years before he – or anyone else – thought about the consequences: that he had broken the rules of classical physics. That he had ushered in a new era: the age of quantum physics.
Planck was only interested in his entropy and the black body. He had cracked the problem, was happy, and turned away from the subject. He liked classical physics and refused to accept quantum physics for a long time. It was not until 10 years after his derivation, when quantum physics had already been made big by others and was on safe ground, that Planck accepted the relevance of quantum physics and eventually the statistical nature of the second rule of thermodynamics.
So is 1900 the year of birth and is Planck the father of quantum physics, when he did not even appreciate it for a long time? If you believe the Nobel Prize Committee: Yes, because Planck received the Nobel Prize in 1918 for the discovery of energy quanta.
But who made the quantum big? Who bravely stood up and spoke of a real discovery instead of a “mathematical trick”? The answer is a stone’s throw away, in the second part about the birthday of quanta.
Do you like what you read? If you don’t want to miss any new posts, don’t forget to subscribe to my blog.
Sources:
Planck’s Route to the Black Body Radiation Formula and Quantization
Max Planck: the reluctant revolutionary