Even scientists are only human. That’s why success stories often read like a comic book: great heroes fighting for the good of humanity. From another perspective, however, it sometimes seems more like the nagging of old white men arguing over who is right. We have already seen a few examples of this: Boltzmann and Planck fighting over entropy, Thomson and Rutherford decoding the atom, Newton and the rest of the world racking their brains over the nature of light. And the Stern-Gerlach experiment, which celebrated its hundredth anniversary this month and which is a milestone of quantum physics, was ultimately the invention of a scientist who desperately wanted to be right.
The Stern-Gerlach experiment was carried out by Otto Stern and Walter Gerlach in Frankfurt on the night of 7 to 8 February 1922. Otto Stern was a Jewish-born theoretical physicist and the brains behind the experiment, who – like so many scientists – left Germany in 1933. Walther Gerlach was an experimental physicist, the “maker” of the experiment, and was… well, if not a Nazi, at least useful to the Nazi regime as “Reichsmarschall für Kernphysik” (Imperial Marshall of Nuclear Physics). This probably contributed to the fact that the Otto Stern Centre at Goethe University Frankfurt only honours one of the two scientists. But perhaps it was also because only Stern was awarded the Nobel Prize for physics in 1943 – without Gerlach.
We already see the first rudiments of human drama here. But the announced squabble was not about dissents between Stern and Gerlach. At the beginning of the famous Stern-Gerlach experiment stood Stern, who thought Bohr’s and Sommerfeld’s atomic model was nonsense.
Bohr’s Atomic Model
It is 1913 and amazingly little is known about the structure of atoms – the basic building blocks of matter (you can find an overview of earlier atomic models here). Only a few years earlier, Ernest Rutherford had substantiated his atomic model with his historic Rutherford scattering experiment: the atom is composed of a positively charged nucleus and negatively charged electrons buzzing around it.
Anyone who knows a little about classical electrodynamics knows that this is utter nonsense! Positive and negative charges attract each other! An atom constructed in this way would be highly unstable. The electron would have to circle into the nucleus and emit radiation with decreasing frequency. But that doesn’t fit, because we already knew that atoms only emit or absorb light at very specific frequencies.
Niels Bohr tackled this problem in a very whimsical way. He set up three postulates that are the foundation for his new atomic model. Postulates are bold assertions that “remain to be proven”. Bohr’s most important (though not explicitly stated) assumption: screw classical mechanics and electrodynamics! I’m doing my thing here! Let’s take a closer look at these three postulates.
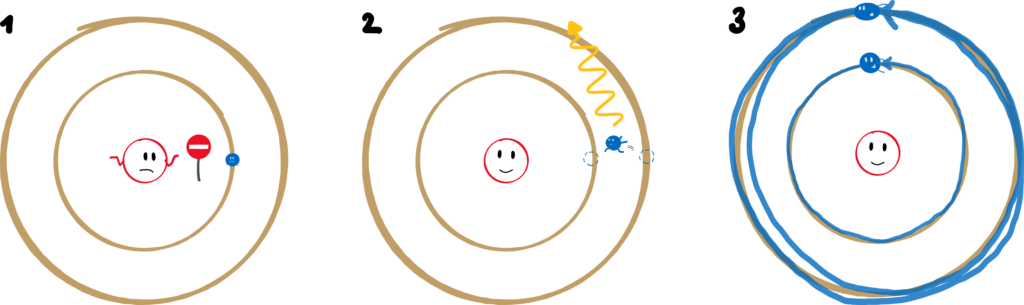
1. An electron can only move along certain orbits. On these, however, it moves radiation-free.
Only certain orbits are allowed? That contradicts classical mechanics: In principle, planets can move in any (elliptical) orbit around the sun. Electrons are only allowed very specific ones – the space in between is taboo. And they move radiation-free in these orbits? We’ve already been through that, it contradicts classical electrodynamics!
2. An electron can jump from one orbit to another (called quantum leap). In doing that, it must absorb or release exactly the right amount of energy.
Okay, if you accept postulate 1, postulate 2 kind of makes sense. If there are only certain, permitted paths, then the electron must hop from one to the other. But the fact that it only absorbs or emits energy in this process – and that in fixed portion sizes – also contradicts electrodynamics. Imagine you could only have 1 A(mpere) of current or 2 A, but not 1.1 A. Absurd.
3. The angular momentum of the electron on the orbit is an integer multiple of Planck’s constant.
That sounds a bit technical, but stay with me! The angular momentum describes, casually speaking, with how much “oomph” something turns. If an electron (metaphorically speaking) flits around the lowest orbit exactly once in a certain amount of time, then it takes exactly twice as long to go around the next higher orbit once. That this minimum angular momentum corresponds exactly to Planck’s constant is very neat and was actually only a guess by Bohr. Or to put it another way: it was precisely this value that made it possible to explain experimental observations with the help of Bohr’s atomic model. As daring as Bohr’s atomic model sounds, it was able to explain many phenomena and make concrete predictions.
The Bohr-Sommerfeld atomic model
The theoretical physicist Arnold Sommerfeld refined Bohr’s atomic model in 1916. Instead of circular orbits, he assumed elliptical orbits, as already known from the solar system. He also introduced three quantum numbers (not just one, like Bohr). Quantum numbers are integers (0, 1, 2, …, -1, -2, …) and the fundament of quantisation: physical quantities no longer lie anywhere on a number ray, but only at these integer steps.
Sommerfeld’s three quantum numbers quantise the energy, the angular momentum and the orientation of the elliptical orbit. Let me repeat that: the ORIENTATION of the orbits! There is not only a restriction on which orbits an electron may take (as Bohr already claimed), but also on how they may be oriented in space. Imagine that on a chairoplane, the seats could only point vertically downwards, upwards, or 90° to the side, but not in between! This phenomenon is called directional quantisation and could explain the behaviour of atoms in magnetic fields.
As a side note: If you look at it from a modern point of view, it is extremely fascinating how well these gentlemen have guessed. Because if you calculate the hydrogen atom once with proper quantum mechanics, you get (almost) the same quantum numbers. But wait, this was not the first lucky guess we will see today.
Stern is not happy
Otto Stern took great offence at this atomic model. Because of the violation of mechanics and electrodynamics, which have existed for centuries? No. Because of the directional quantisation of the electron orbits. He wanted to put an end to this nonsense and come up with an experiment that disproved directional quantisation.
No sooner said than done. He needed comrades-in-arms: Walther Gerlach, who was fit in experimenting, but also Max Born and the mechanic Adolf Schmidt. And they needed a lot of donations to be able to set up the experiment, which came from Albert Einstein and various companies, among others. In February 1922, everything was ready to go.
Mini-magnets in a magnetic field
To prove (or disprove) the directional quantization, Stern and Gerlach used a well-known law of electrodynamics. It refers to the behaviour of magnetic dipoles – sort of elementary mini-magnets – in a magnetic field. The principle of a compass is that the needle (a mini-magnet) aligns itself in an external magnetic field (the earth’s magnetic field). However, the needle only rotates: if you were to build the compass on a small cart, it would not drive to the North Pole. However, this is different in an inhomogeneous, i.e. a non-uniform, magnetic field. If the magnetic field at the Earth’s north pole, for example, were much stronger than at the south pole (for example, if the Earth were not a sphere but an ice cream cone with the tip pointing upwards), the little compass cart would travel to the north pole!
Why is that? Let’s draw the north pole red and the south pole blue (picture below). Since the north pole is stronger, the upper part of the magnetic field is “redder” than the lower part is blue. If a mini-magnet (i.e. a magnetic dipole) flits through this field and its south pole points upwards (left panel), it will be deflected upwards. This is because the north and south poles of magnets attract each other. Because the magnetic field is stronger at the top than at the bottom, the red part of the field attracts the blue part of the magnet more strongly than the blue part of the field attracts the red part of the magnet. The reverse is true if the north pole of the mini-magnet points upwards (right panel). Then the red part of the field repels the red part of the magnet more strongly than the blue part of the field repels the blue part of the magnet.
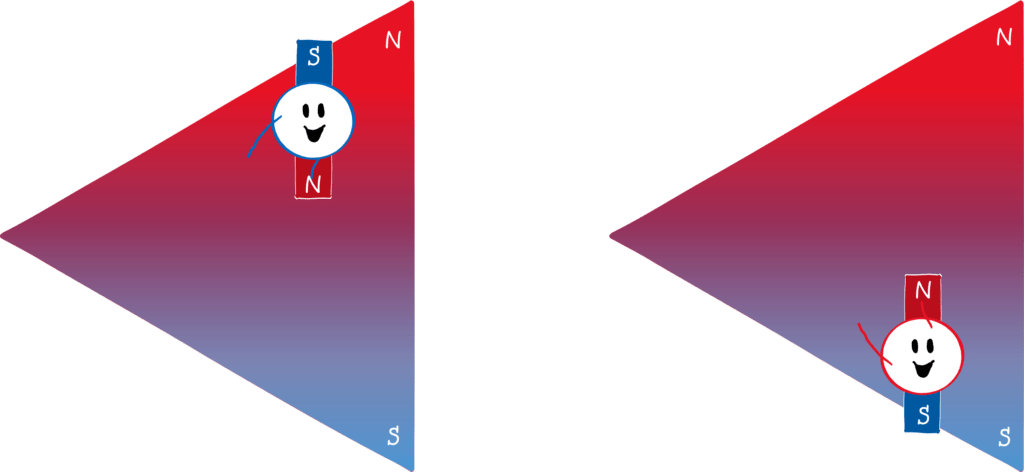
The Stern-Gerlach experiment
The key of the Stern-Gerlach experiment is that atoms behave like mini-magnets. Classically, one would expect that the mini-magnets in the atom can point in any direction. If you shoot atoms through an inhomogeneous magnetic field, you should see a smeared stripe on the opposite side.
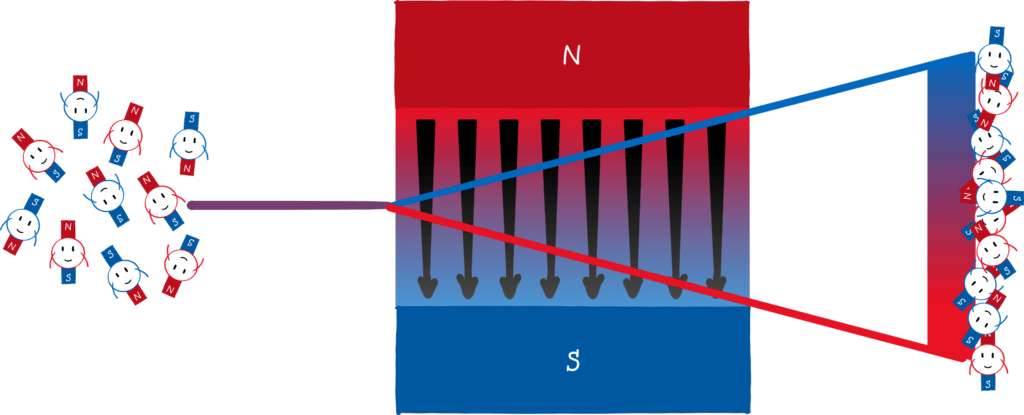
According to the Bohr-Sommerfeld atomic model, however, the direction of the orbits, and thus the direction of the mini-magnets, is quantised! So instead of one stripe, you would see two (or more) points on the screen.
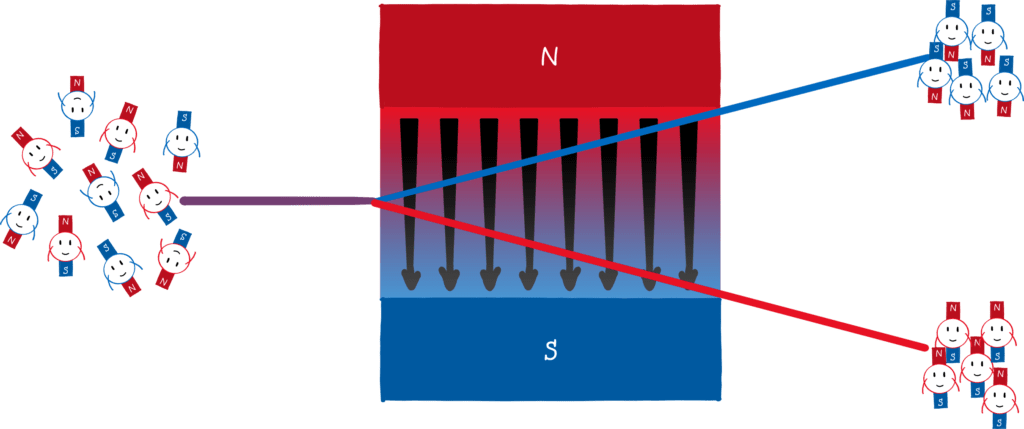
That’s exactly what Stern and Gerlach did in their experiment with silver atoms and what came out? Drum roll! Two points! Stern was so pleased that he almost forgot that he was originally against directional quantization. He wrote an excited telegram to Gerlach with the message “Bohr is right!”. Stern is an exemplary loser. He wanted to refute Bohr and in the end, he substantiated his atomic model.
Appearances are deceptive
However, one small detail is still not right. It was later found out that the outermost electron of silver had an angular momentum of zero. You cannot “quantise” an angular momentum of zero – it is always zero. Stern and Gerlach originally assumed that the angular momentum was 1, so it could have pointed upwards (1), downwards (-1) or towards the centre (0). But the fact that two, and not the expected three, points were seen in the experiment apparently didn’t really bother anyone. But with an angular momentum of 0, there are no mini-magnets. So one should not observe any deflection of the atoms at all. It took three years to resolve this puzzle.
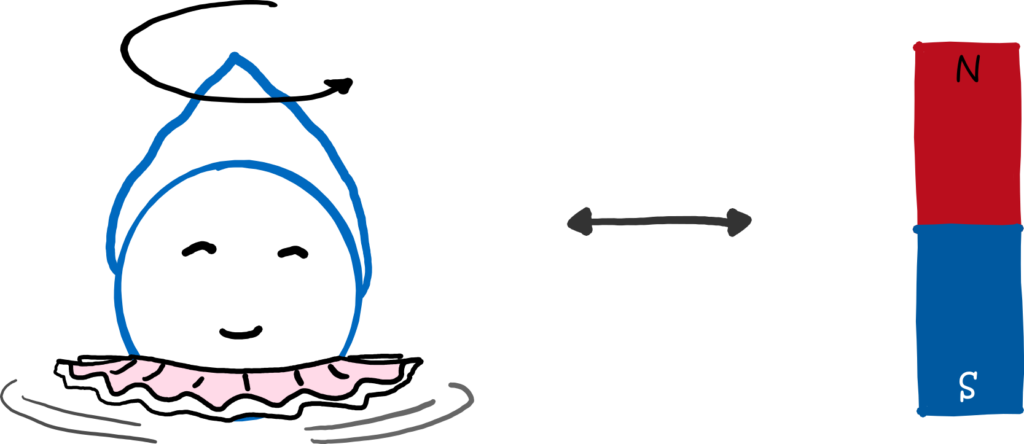
The angular momentum of the electron in its orbit around the atomic nucleus is not the only important one in an atom. Beyond that, the electron itself rotates – at least metaphorically speaking. The electron has a spin, which we like to think of as intrinsic angular momentum. Because of this spin, the electron has a magnetic moment and behaves like a mini-magnet. And this angular momentum has the value: 1/2! This means that it can point either upwards (+1/2) or downwards (-1/2) and that is exactly where the two points in the experiment come from.
So in the end, Stern and Gerlach did not show (let alone disprove) the directional quantisation of the orbital angular momentum of atoms. They have observed the directional quantisation of the electron’s spin – something completely different. So they were absolutely lucky that the outcome of the experiment was so similar to what they expected at all.
Lucky hit or not – the Stern-Gerlach experiment made history. Prizes and buildings were named after these gentlemen. The basic idea behind the experiment, i.e. the experimental setup, is still used today in an advanced form for many technologies. Among them are nuclear magnetic resonance (NMR) and magnetic resonance imaging (MRI). What do we learn from this? Sometimes, all you need is a good dose of luck and a bit of childish defiance, and something good might come out of it!
Do you like what you read? Then you can buy me a coffee here! And if you don’t want to miss any new posts, don’t forget to subscribe to my blog.